Research topics
- Combining live cell imaging and single-cell RNA sequencing
- Three-dimensional ultrafast live imaging of intracellular microstructure
- Large-scale automatized collection of data pairs of Raman Spectrum-RNA Sequence in a single cell
- Combining super-resolution imaging of actomyosin organization and machine learning to identify mechanicalstates of endothelial cells in developing blood capillaries.
- An imaging toolbox for chromosome identification in live mouse oocyte and embryo
- Development of the method to grasp the distinct Cell-states using NMR
- Investigation of cell specificity responsible for MET events within kidney organoids using machine-learning approaches
- Stem cell lineages of the complex brain and their underlying principle
- Nano fluidic chip -From micro to nano-
- Developing single-molecule bioanalysesto detectand distinguish intracellular molecules.
- Single cell analysis of human iPS-derived retinal organoid
- Exploration of a novel tissue stem cell population and niche
- Decoding the mechanism of neural epithelium formation during the development of neural organoids.
- Prediction of aging status using image data
Combining live cell imaging and single-cell RNA sequencing
Summary
Recent technologies in next generation sequencing enable us to analyze whole genome expression in individual cells. However, analysis of whole genome expression in a cell basically destroys the cell. If we can predict the whole genome expression only by observing the cell, we can understand the details of internal cell state while keeping the cell alive. Toward this goal, we connect massive amounts of single-cell imaging data with single-cell genome expression data using machine learning.
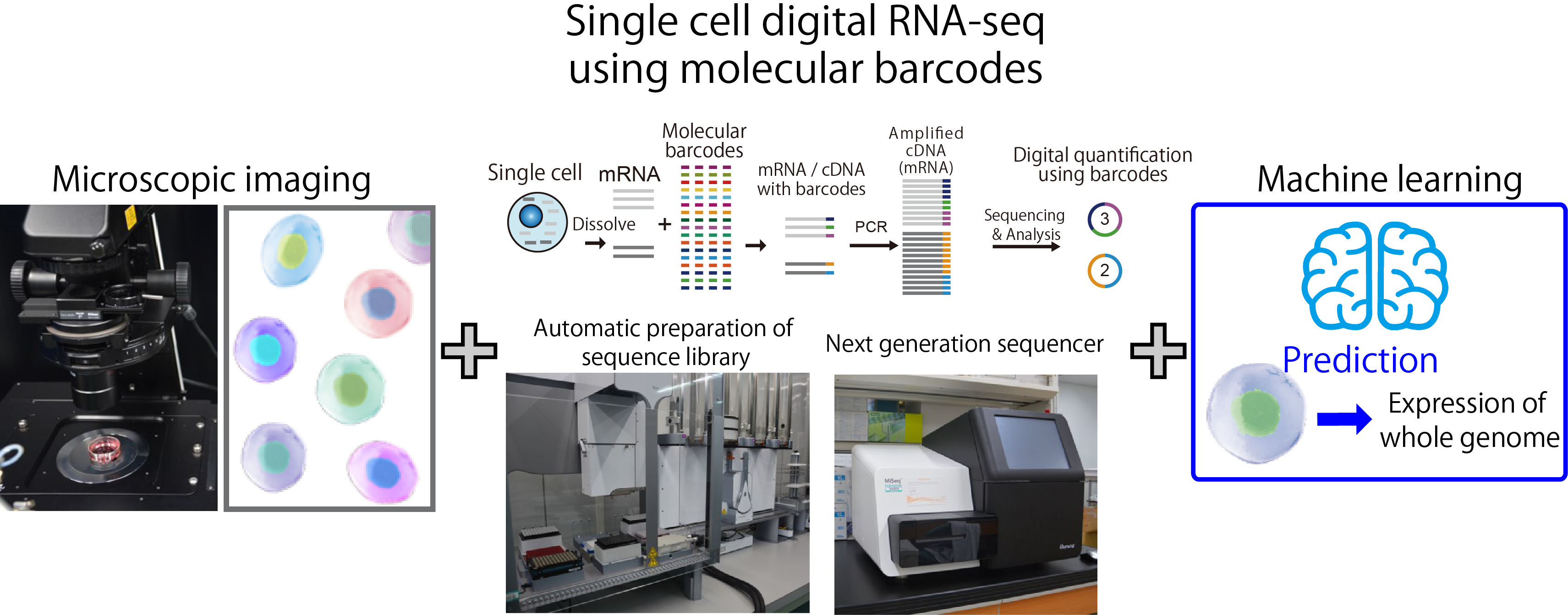
Click to enlarge
Related labs
Three-dimensional ultrafast live imaging of intracellular microstructure
Summary
We observe the microstructure in the living cell with the world's highest shutter speed by using a uniquely developed "ultrafast super-resolution microscope". We DECODE the state of living cells noninvasively by detailed observation of morphology and dynamics of subcellular organelle such as mitochondria as well as microstructure in the nucleus.
Outer membrane of mitochondria captured by super-resolution microscope
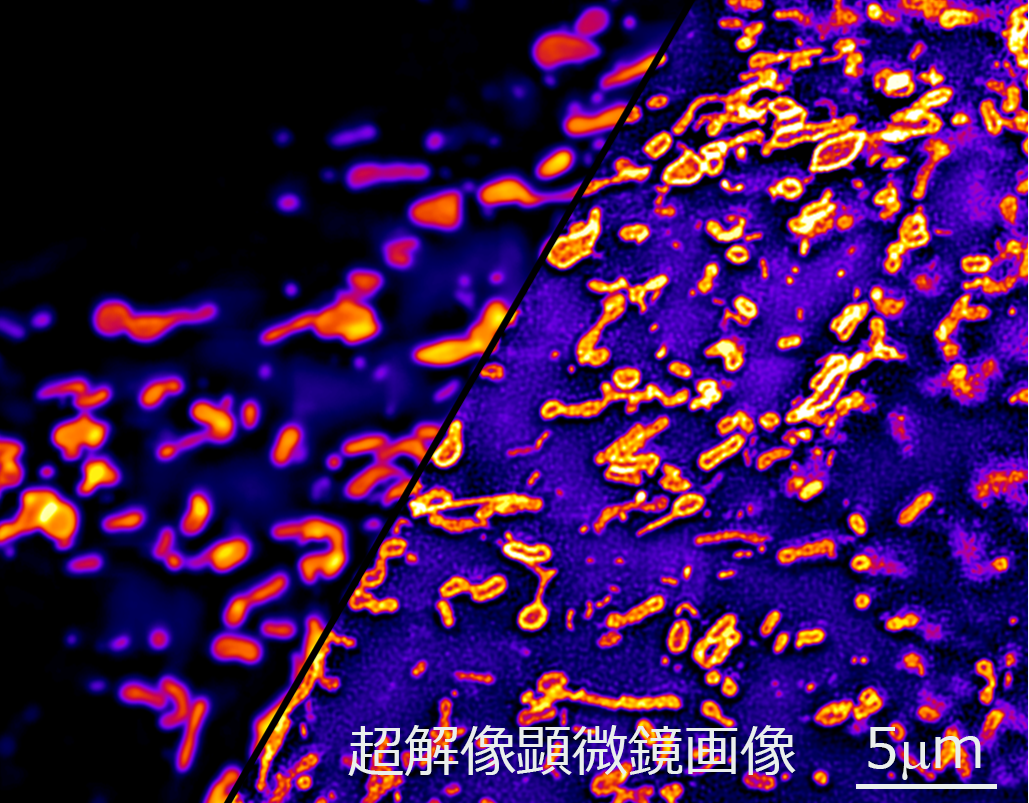
Super-resolution 3D live imaging of endoplasmic reticulum
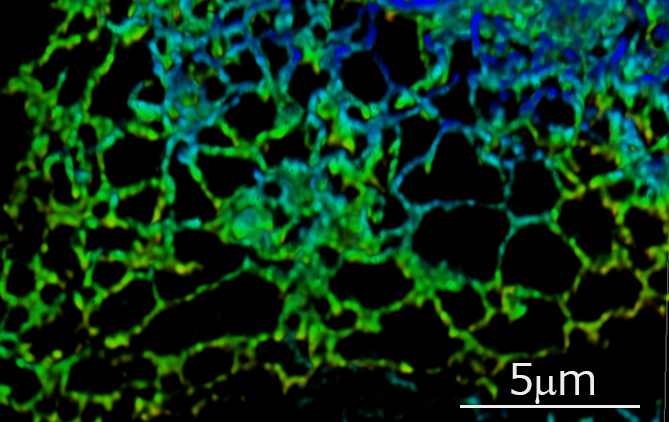
Related laboratory
Large-scale automatized collection of data pairs of Raman Spectrum-RNA Sequence in a single cell
Summary
The aim of this project is to establish a technology for predicting gene expression from Raman scattering spectrum emitted from a cell so as to conceptively demonstrate how to "predict the intracellular information from scattering light". The Raman spectrum from a cell is determined by combinations of all molecular vibrational modes (CC, SH, benzene ring, etc.) inside the target cell. Since a cell is composed of various molecular species, the Raman spectrum will be too complicated to be decomposed. However, no matter how complex it is, if gene expression changes in the cell, the types and ratios of constituent molecules will change, and the Raman scattering spectrum is expected to change accordingly. In other words, the measured Raman spectrum is indirectly but surely correlated with the gene expression pattern via cellular function and/or state. From complexly modified Raman scattering light that can be measured non-invasively, it is possible to predict the gene expression that can only be measured invasively. To demonstrate the above concept, we collected data pairs of Raman spectrum and RNA sequence of 10 drug-resistant E. coli, and constructed a machine learning model to connect these two data pairs. With this machine learning model, we have succeeded in estimating the gene expression pattern of drug-resistant E. coli from the Raman spectrum with an accuracy of 80 to 90%. Furthermore, in order to demonstrate that the same concept holds for mammalian cells, we are carrying out the same experiment using disease-derived induced pluripotent stem cells (iPS cells). In addition, we are constructing a microscope system that enables automatic collection of Raman spectrum-RNA sequence data pair in a single cells.
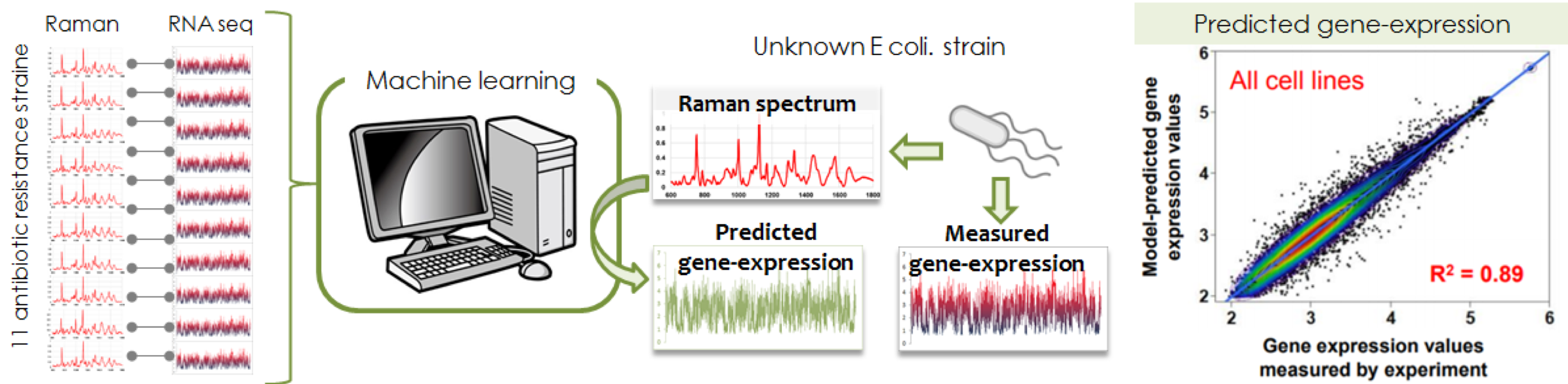
Click to enlarge
An imaging toolbox for chromosome identification in live mouse oocyte and embryo
Summary
Blood capillaries supply nutrients to tissues and carry away metabolic waste. New capillaries can be generated by sprouting angiogenesis, a process during which endothelial cells (ECs) migrate from pre-existing blood vessels to form new sprouts that are later perfused with blood flow. By using machine learning-based labelling, we observed that ECs of zebrafish embryos undergo extensive migration within the capillaries immediately after perfusion with blood flow. Furthermore, using Super Resolution Spinning Disk Microscopy we observed that acto-myosin networks in these ECs vary considerably from one cell to another, indicative of different mechanical states. Interestingly, one day later EC motility is substantially reduced and so is the acto-myosin network diversity observed, suggesting a transition of ECs and capillaries into a more stable state. We are now trying to understand the link between different actomyosin organization and EC state (migrating, elongating, constricting, or static) to provide a description of the steps involved in capillary stabilization. Our study provides unprecedented resolution on blood vessel capillary formation in vivo, allowing identification of novel mechanisms involved in tissue vascularization.
An imaging toolbox for chromosome identification in live mouse oocyte and embryo
Summary
Multiple chromosomes with different sizes and gene densities exist in cells. Chromosomes are evenly segregated by cell division. However, in oocytes and embryos, the risk of miss-segregation of specific chromosomes increases with aging. So far, there is no tool that enable us to analyze the dynamic behavior of individual chromosomes in live oocytes and embryos. In this study, we develop an imaging tool that identify all of individual chromosomes in live mouse oocytes and embryos, and clarify the mechanism of miss-segregation. Using inactive Cas9 and multiple types of fluorescent proteins, specific marks was labeled on all chromosomes, and the marks are analyzed by machine-learning-based prediction program.
Development of the method to grasp the distinct Cell-states using NMR
Summary
Cells represent various distinct states appropriate for the processes of cell-division and/or
cell-differentiation, and for surrounding environment. If such various cell-states can be detected as not
only the morphologic changes of cells but also the numeric differences of something, that is very helpful
for understanding of the detail mechanisms of cell-cycle and environmental acclimation, and for optimizing
of the cell-differentiation condition to get the specific organs. NMR can detect molecular differences and
its quantities by observation of the intrinsic magnetic properties of the atomic nuclear without any
cell-damages. In addition, together with our cell-encapsulation technique, we can achieve the NMR
observation for living cells over a long period.
Since cells synthesize / degrade / import / export
various molecules depending on their cell-states, we are challenging to develop the method for temporally
and quantitatively grasping the various distinct cell-states by the combination of NMR observation and
data science techniques.
Investigation of cell specificity responsible for MET events within kidney organoids using machine-learning approaches
Summary
During kidney development, nephron progenitor cells develop into the nephron, a functional unit of the
kidney, via mesenchymal-epithelial transition (MET). We previously established an in vitro system in which
METs can be artificially induced to human iPSCs-derived nephron progenitor cells by the stimulation of
canonical-Wnt signalling. Interestingly, we further demonstrated that those MET events occur constantly
and singularly within a uniform nephron progenitor population.
Here, in this research project, we aim
to elucidate the mechanism that control such rare MET events using an in vitro MET occurrence system. For
this purpose, we will construct a system for real-time monitoring of MET events, and then use a machine
learning system to prospectively detect cells just before the onset of MET and analyze their
characteristics.
This study will help to elucidate the mechanism of determining the number of nephrons
in the human kidney, and the findings can be applied to the development of a new method for efficient
artificial induction of kidney tissue in the future.
Reference
- 1. *Takasato, M., Er, P.X., Chiu, H.S., and Little, M.H. (2016). Generation of kidney organoids from human pluripotent stem cells. Nature Protocols 11, 1681–1692.
- 2. *Takasato, M., Er, P.X., Chiu, H.S., Maier, B., Baillie, G.J., Ferguson, C., Parton, R.G., Wolvetang, E.J., Roost, M.S., Chuva de Sousa Lopes, S.M., et al. (2015). Kidney organoids from human iPS cells contain multiple lineages and model human nephrogenesis. Nature 526, 564–568.
- 3. Takasato, M., and *Little, M.H. (2015). The origin of the mammalian kidney: implications for recreating the kidney in vitro. Development 142, 1937–1947.
Stem cell lineages of the complex brain and their underlying principle
Summary
Cell lineages of neural stem cells are highly diverse in gyrencephalic brain formation, in contrast to stereotypic ones in rodents. This heterogeneity in lineages associates with the expansion of brain size and complexity. We are trying to see whether there is a hidden pattern in this heterogeneity by determining and analyzing a large number of neural stem cell lineages.
Nano fluidic chip -From micro to nano-
Summary
We have many technologies on microdevice development for rapid and high-throughput analysis of cells and
has applied these technologies to life sciences. In BDR, we have fabricated and provided many microdevices
for cell and tissue analysis to many laboratories or projects. Recently, this concept has been extended to
molecules, namely nano scale and by combining with technologies for molecular measurement, simulation, or
analysis in BDR, we aim to pioneer a new scientific field.
However, nanoscale channels are not only
difficult to be fabricated, but also difficult to flow liquid in them. The scale is just between the
molecules and bulk. So, it was suggested that water and other molecules behaviors are different from bulk.
This field is difficult area not only as engineering but also science, but results with large impact is
expected.
The final goal of this project is to construct a platform to manipulate single molecules in
nanoscale. Before that, we aim to establish fundamental technologies including fabrication, fluid control,
and measurement method in nanochannel with Okada TL.
Developing single-molecule bioanalysesto detectand distinguish intracellular molecules.
Summary
Taking advantage of single-molecule imaging, we aim at developing ultrasensitivebioanalyseswith a single-molecule sensitivity to detect and distinguish various biomolecules originating from a cell.The key of our strategy is the exploitation of our home-built light-sheet microscope, the PISA microscope, which can perform 3D single-molecule imaging with high-speed. Such aspects are particularly useful for systematic studies about gene expression at single-molecule level and ultrasensitive medical tests using body fluids. As various advantages of the PISA microscope in biological experiments, such as versality, high-throughput, and ultrasensitivity,were highly evaluated, a top-tier global optics company released a new microscope in 2020 that employed the design of the PISA microscope (ZEISS Lattice Lightsheet 7). In the DECODE project, we are developing several new typesof bioanalysis for single-cell study and medical applications by combining conventional biochemical analyses, 3D single-molecule imaging, and imageanalysistechnique.
Single cell analysis of human iPS-derived retinal organoid
Summary
In November 2020, clinical research for the treatment of photoreceptor degeneration using neural retina sheets induced to differentiate from human iPS cells began. The neural retinal sheet used in this study was researched and developed at our institution, but at present, the retinal sheet for transplantation ismanually cut out fromtheneural retina toremove pigment epithelium and ciliary body.Butnot only does it require skillful techniques to cut out, but the size that can be cut out is as small as about 1 mm x 1mm, and many neural retinalsheets are required to cover a wide range of lesions, which requires enormous cost and labor. One solution is to develop a large neural retinal sheet. However, the technique for producing a largeretinal organoid that ensure qualityandsafetyhas not been establisheddue to the difficulties of controlling the differentiation from spatially and temporally heterogeneous cells.Therefore, in this project, we aim to develop a large-scale next-generation transplantation retinal sheet that suppresses differentiation into cells other than photoreceptor cells by reconstructing the retinal sheet only from retinal progenitor cells in a specific differentiation stage. Single cell (sc) RNA-seq is performed to understand the cell characteristics of retinal progenitor cells necessary for that purpose.
Exploration of a novel tissue stem cell population and niche
Summary
The tissue stem cells are the most important cell population in adult tissueshomeostasis. In particular,
the self-renewal and differentiation capacities of tissue stem cells are indispensable for tissue
metabolism and regeneration from damage. The airway epithelium is exposed to invasivebacteria, viruses,
and chemical stimulus thatmay damage the tissue. Airway epithelium has a relatively slow metabolism under
normal conditions, but upon injury, cells proliferate rapidly to repair the damaged area. This type of
tissue regeneration is called facultative regeneration in which stem cells activate their self-renewal
capacity in response to injury. Multiple tissue stem cell populationshave been identified in airway
epithelium that expected to respond to various type of damages to protect pulmonary system.
Recent innovations in stem cell culture techniques have made it possible to reconstitute mini-organs,
called organoids, by culturingadult tissue stem cells for long periods of time in the laboratory. In lung
organoid culture, lung tissue stem cells are isolated and grown into spheres by culturing them on a
three-dimensional scaffold, which is suitable for studying lung stem cell dynamics. In this research
project, we seek novel stem cells by combining scRNA-seq analysis with image analysis of lung tissue stem
cells and organoids, which will be performed in collaboration with the Dr. Shiroguchi’s Laboratory. The
candidates of the novel stem cell markers will be characterized using mouse lungs. In particular,in
vivostem cell properties will be verified based on cell dynamics during metabolism and injury
regeneration.
Decoding the mechanism of neural epithelium formation during the development of neural organoids.
Summary
Neural organoid is the three-dimensional neural tissue that is derived from pluripotent stem cells (PSCs). It is elusive how the neural epithelium develops during self-organizing formation of neural organoids. In this research subject, we will first induce neural organoids using knock-in human PSCs that can determine the generation of neural epithelium, and then perform 4D imaging to capture how the epithelial structure is constructed. Finally, we will elucidate the genetic background of the self-organizing formation of epithelial structure by single-cell RNA-seq analysis at the key point of the process. Combining the information of imaging and RNA-seq, it will be possible to explain how the complexed epithelial structure emerge.
Prediction of aging status using image data
Summary
Aging is a biological process that occurs over an entire lifetime. Because aging is a rather complex process that takes a long time and is sensitive to genetic and environmental factors, a comprehensive understanding of the aging process remains unsolved. This research plan aims to comprehensively understand the aging process by monitoring changes in appearance, gene expression, metabolism, and muscle function over an entire lifetime using a genetically homogeneous model organism C.elegans. Furthermore, we examine the links between obtained data using the machine learning approach. This study would help us deepen our knowledge of the aging process and might predict the state of aging of an individual animal based on its appearance.